suspended growth (activated sludge) process (page 2)
nitrogen removal
In UWW and in many IWW, nitrogen can be found mainly in its organic form and as ammonia. In a plant designed for nitrogen removal, this removal is the end result of four operations (figure 12).Other ways are under development:
- ammonification: the conversion of organic nitrogen into ammonia nitrogen;
- assimilation: ammonia used to synthesise living matter that will be eliminated with the excess sludge;
- nitrification: oxidation of ammonia nitrogen into nitrites and then into nitrates;
- denitrification: converting nitrates into nitrogen gas that is discharged to the atmosphere.

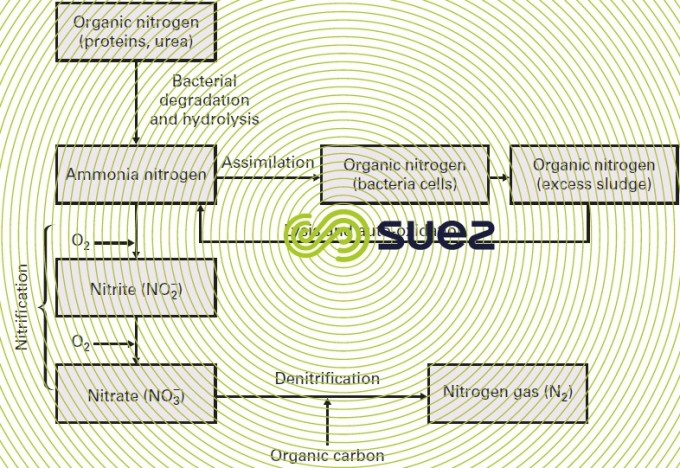

ammonification
Organic nitrogen is converted into ammonia nitrogen. The ammonification rate is identical to the rate at which carbonaceous pollution breaks down.
In most cases, given the nature of organic nitrogen and of wastewater treatment plant operating parameters, and more especially, water contact time in the structures, most of the organic nitrogen will easily be ammonified.
assimilation
Assimilation is where some of the ammonia nitrogen is used by the bacterial synthesis function. Assimilation can play a crucial role in nitrogen removal from some IWW when the

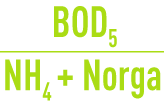
ratio is high. As a rough guide, nitrogen removed by excess sludge can be assumed to represent from 5 to 10% of the excess sludge.
nitrification
Nitrification is the biological process that is carried out in two stages by specialised autotrophic micro-organisms:
- NH4+ is oxidised to produce nitrites NO2-; this is mainly carried out by Nitrosomonas type bacteria with the following reaction
- NO2- is oxidised to produce nitrates NO3-; the bacteria responsible for this second reaction mainly belong to the Nitrobacter genus
hence the total oxidation reaction


Based on these reactions, the oxygen required for the complete oxidation of ammonia nitrogen will be 4.57 g O2 · g–1 oxidised N-NH4 (excluding the nitrogen used for synthesis).
The following equation can be used to estimate the quantity of alkalinity required:


I.e. 7.14 g of alkalinity (expressed as CaCO3) or even 0.714 °F per g oxidised N-NH4. Additionally, approximately 0.17 g of new cells will be produced per g of N-NH4.
growth rate and sludge age
The growth rate for Nitrosomonas and Nitrobacter bacteria is slow compared to that of heterotrophic organisms. As a rule, it is the most limiting parameter when dimensioning the nitrification reactor. More specifically, the limiting phase is the oxidation of ammonium to produce nitrites, associated primarily with the activity of Nitrosomonas (excluding individual transient episodes such as commissioning the plant).Given the low growth rate, the capacity of a biological reactor in a plant designed to remove nitrogen will often be dictated by aerated sludge age. In effect, the quantity of nitrifying bacteria appearing in the system must be equal to or greater than the quantity lost in the excess sludge extracted, otherwise "leaching" will occur.
Leaching is very temperature-dependant. Under pH conditions of between 7.2 and 8, the link between the minimum age of aerated sludge required to maintain stable nitrification and temperature is shown by the curve in figure 13.

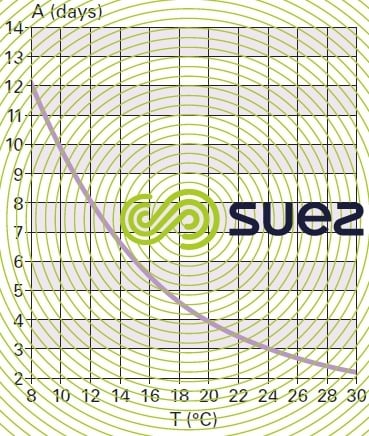

This curve shows that at a temperature of 12°C, the temperature often used for dimensioning purposes, nitrification compels us to consider sludge over 8 days old, requiring the use of low loading processes. Below 8°C, nitrification initiation is very haphazard. On the other hand, if the nitrifying fauna can be developed in advance at a normal temperature, the nitrification process can be continued at lower temperatures with lower N-NH4 oxidation efficiency because nitrifying bacteria leaching will only take place gradually. Similarly, nitrification can be maintained even with sludge younger than the minimum age providing that this operating method is only applied for a limited period of time (rainfall event, for example).
In some applications (influent having a low C/N ratio), we need to check that the capacity of the aerated reactor dictated by sludge age is compatible with maximum nitrification kinetics (at the temperature concerned).
factors that affect nitrification
Nitrification is affected by a range of environmental factors including pH, dissolved oxygen concentration, the presence of inhibitor or toxic elements.
- pH and alkalinity: nitrification is pH-sensitive. Nitrification will be optimum for a pH range of between 7.2 and 8.0 and will decrease significantly at pH levels below 6.8. The reaction consumes alkalinity and, in some soft water areas, alkalinity has to be added to waste water in order to achieve an acceptable pH (more especially when nitrification is combined with simultaneous phosphorus precipitation). The reagents that can be used for this purpose are lime, sodium hydroxide or bicarbonate of soda;
- dissolved oxygen: nitrification kinetics are affected by the concentration of dissolved oxygen in the mixed liquor. These kinetics will increase up to concentrations of 3 to 4 mg · L–1; it will be strongly inhibited for concentrations below 0.5 mg · L–1. The recommended operational set point figure will be approximately 1.5 to 2 mg · L–1 of dissolved O2.However, for the same set point figure, different nitrification kinetics can be recorded from one site to another because the dissolved oxygen depends on the size of the biological floc and on the mixed liquor’s total oxygen demand. In effect, oxygen from the liquid phase diffuses through the floc and the bacteria that are the furthest from the periphery will be exposed to increasingly weak concentrations;
- inhibiting and toxic elements: nitrifying organisms are sensitive to many organic or mineral compounds at concentrations far lower than those that affect heterotrophic aerobic bacteria.
- The main mineral toxins (copper, zinc, nickel, hexavalent chromium, cadmium, mercury) will be inhibitors from a concentration of only a few mg/l. This will vary depending on the compounds. Bacteria will also be sensitive to the variations in concentration of these elements. For example habituation at concentrations of between 10 and 20 mg /l could occur over a long duration for some of these metals.
- Salinity, and namely variations in salinity, will also have an inhibiting effect on nitrification.
- For organic compounds, it is necessary to distinguish between biodegradable organic compounds (phenol for example) which will be toxic in the event of a build-up in the reactor or during high load variations and organic compounds which are little or not at all degradable and which may be inhibitors at very low concentrations : 0.076 mg/l for thiourea for example.

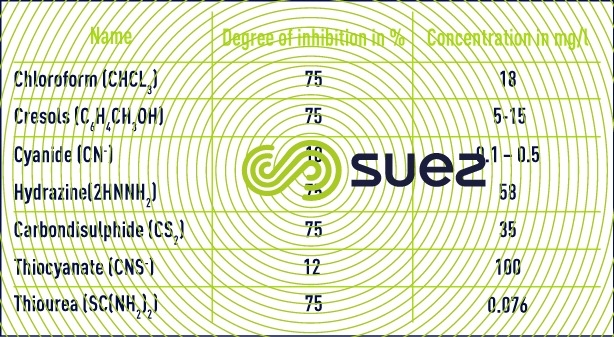


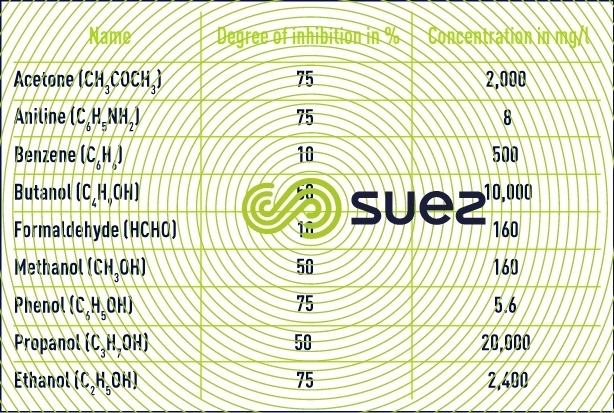

It should also be noted that the presence of biodegradable organic matter will decelerate ammonium oxidation as the result of competition from heterotrophic organisms for dissolved oxygen and that this situation will even occur when high O2 concentrations are maintained (3-4 mg · L–1). That is why a number of authors have stated that nitrification assumes that carbonaceous pollution has been satisfactorily removed before hand; in effect, a degree of overlap is possible but will not apply to high loading.
denitrification
Denitrification is the process whereby some bacteria will reduce nitrates to form nitrogen gas. Biological denitrification is an integral aspect of biological nitrogen removal, combined with the nitrification stage, designed to satisfy the discharge standard for total nitrogen. Indeed, biological systems may involve two nitrate reduction modes; the assimilatory method and the dissimilatory method, the latter being performed by either autotrophic or heterotrophic bacteria.
The dissimilatory method is the most widely applied and uses heterotrophic bacteria. Nitrate reduction takes place through various reactions that can be indicated schematically as follows:


This is an anoxic process (that takes place in the absence of oxygen but in the presence of the oxidised form of nitrogen) and requires an electron donor. The electron donor should preferably be biodegradable COD found in raw water; failing this COD. Organic matter from the biomass (endogenous respiration of the sludge) or via a source of exogenous carbon such as methanol or an acetate can also be used.
Unlike nitrification, the heterotrophic denitrification reaction provides alkalinity that represents 3.57 g (expressed as CaCO3) per g of reduced N-NO3.
growth rate
In biological denitrification, the kinetic equations used to describe bacterial growth and substrate use are similar to those described for heterotrophic aerobic bacteria. The main difference comes from the fact that the nitrate acts as the electron acceptor instead of oxygen.
The effect of temperature on the rate of denitrification is similar to that used for BOD break down in an aerobic environment (figure 14).
Nevertheless, some parameters remain specific to denitrification; dissolved oxygen, the source of organic carbon and, to a lesser extent, pH.

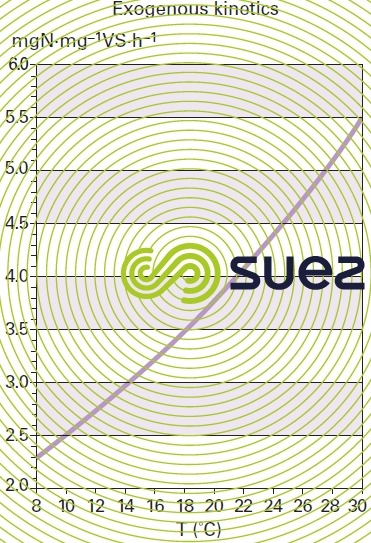

factors that have an effect on denitrification
- pH and alkalinity: given that alkalinity is produced during denitrification reactions, pH remains generally high. The optimum pH range will be between 7 and 8.2:
- dissolved oxygen: the presence of dissolved oxygen inhibits denitrification. In effect, denitrifying bacteria obtain their energy from the energy that is released when electrons are transferred from organic compounds to O2, NO2- or NO3-. If these three elements are available to act as electron acceptors, the final acceptor chosen will obviously be the one that delivers the greatest amount of energy per unit of oxidised organic matter. As this amount of energy is higher for oxygen than for other cases, electrons will be transferred to oxygen as a priority (more denitrification). In practice, it is hard to specify limit concentration levels for O2 beyond which denitrification will be inhibited because these values depend on the size of the floc, on the nature and concentration of the organic carbon (see suspended growth (activated sludge) process). However, dissolved O2 concentrations above 0.3 mg · L–1 already provide significant inhibition. Another approach consists in measuring not the dissolved O2 but the oxidation reduction potential in relation to the hydrogen electrode (HE).Although the figures obtained do not agree, it can be said that denitrification will only occur under acceptable conditions when the oxidation reduction potential is below 200 mV;
- source of carbonaceous substrate: due to its nature and concentration, the carbonaceous substrate has a major impact on denitrification kinetics. The rapidly biodegradeable carbon contained in raw water will permit denitrification to occur at high kinetic unlike carbon which biodegrades slowly. In certain processes, sulphides can also serve as a substrate for denitrification. An exogenous source of carbon, such as methanol or acetate, can be added during pre-anoxic or post-anoxic in such a way as to provide the BOD necessary to reduce nitrates and significantly increase denitrification kinetics.
- Mass loading / sludge age: We also note that an increase in the bioreactor’s mass loading has a positive effect on the kinetic of denitrification. This effect is often little used owing to the fact that nitrification /denitrification facilities are limited to operate at low load to ensure nitrification. However, certain processes or particular set-ups (mixed cultures, "linked" cultures) can benefit from this advantage.
Various basic schemes are used to achieve denitrification, resulting in variable kinetics.
The first scheme is an anoxia digestion tank followed by an aeration tank where nitrification takes place (figure 15 (a), a scheme designated Modified Ludzack-Ettinger or "inlet anoxic").The nitrates produced in the aeration tank are recycled in the anoxic tank. The raw water’s organic substrate provides the electron donor for oxidation reduction reactions that use nitrates. The denitrification reaction is called "pre-anoxic denitrification". This is a fast reaction but it is limited by the quantity of NO3- injected by recirculation.
In the second scheme (figure 15 (b)), denitrification takes place after nitrification and the electron donor source comes exclusively from endogenous respiration. The denitrification reaction is called "endogenous denitrification"; the kinetics involved are markedly lower than the preceding denitrification because the influent’s BOD has been consumed and is no longer available as an energy source. We can note that this configuration is not used to treat urban wastewater effluents.
The third scheme (figure 15 (c)) consists in introducing aerated periods for nitrification and anoxic periods for denitrification within the same reactor by implementing sequenced aeration and continuous mechanical mixing. Denitrification is called "combined denitrification" and the value for the kinetics achieved will fall halfway between the two previous values.

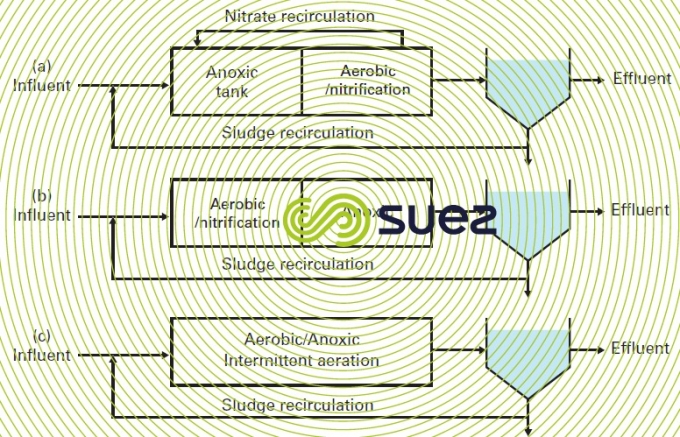

Other configurations can be envisaged, namely the tri-zone process. In this configuration, a so-called "endogenous" zone is added downstream of the anoxic zone and the aeration basin.

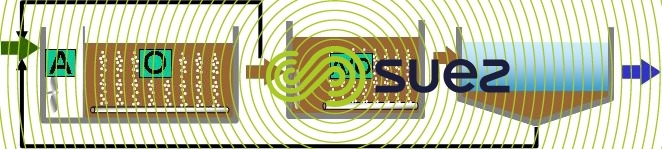

This configuration presents several advantages, including:
- high denitrification performances are obtained;
- reduction in the rate of internal recirculation owing to the fact that the recycled liquor has a higher nitrate concentration than that of the water treated.
anammox bacteria
The Anammox reaction (AnNaerobic AmmMMoOnium OxXidation), predicted as early as the 1980s and discovered at the beginning of the 1990s, offers an alternative to conventional nitrogen treatment. Today, we consider that these bacteria are ubiquitous; they are present as much in the oceans, where they are responsible for between 30 and 50% of nitrogen consumption, as in fresh water aquatic eco-systems (estuaries, soils…).
During this reaction, in anoxic conditions (in the absence of oxygen), ammonium is transformed into gaseous nitrogen using nitrites as electron acceptors, according to the following reaction:


We can note that a fraction of the nitrogen is found in the form of nitrate following this reaction.
The pre-requisite for this reaction is partial nitrification, in other words, the oxidation of the ammonium to nitrites. This corresponds to the first stage of conventional nitrification and it makes use of « classic » nitrifying bacteria.
Conventional nitrification is ensured in two stages:
- The oxidation of ammonium to nitrite, ensured by bacteria of the Nitrosomonas, AOB type (AOB :Ammonium Oxidizying Bacteria)
- The oxidation of nitrites to nitrates, ensured by bacteria of the Nitrobacter, NOB type (NOB :Nitrite Oxidizying Bacteria)
As represented below (figure 17), one of the elements encouraging partial nitrification is that at a high temperature (higher than 18°C), the growth rate of AOB is higher than that of NOB, which limits the development of the latter.

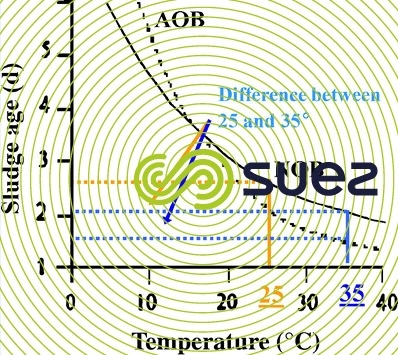

Generally speaking, we consider that partial nitrification is favoured by:
- A high temperature (higher than 22°C);
- A low residual oxygen concentration during the aerated phase (less than 1 mg/L);
- A pH of between 7 and 8.
The Anammox reaction requires:
- The presence of two substrates (nitrite and ammonium) at a ratio close to 1.3;
- A moderate nitrite concentration ; there is no limit value accepted by all insofar as bacteria have a great capacity to adapt, but a concentration exceeding 100 mg/L (in N/NO2) can be considered as a limit value;
- The absence of residual oxygen and biodegradeable carbon.
The deammonification reaction (partial nitrification + Anammox) was mastered at the industrial stage at the end of the 1990s for the treatment of nitrogen from centrates resulting from the dehydration of anaerobically digested sludge. This effluent, which is concentrated in nitrogen and lacking in biodegradeable carbon, is also a favourable element for the growth of these autotrophic bacteria (slow growth). The first tests and industrial applications have been conducted on this type of effluent, which permitted more economical nitrogen treatment from the beginning of 2000.
The Cleargreen process was developed to treat centrates resulting from the dehydration of anaerobically digested sludge. Partial nitrification and autotrophic denitrification reactions occur to a varying degree in a SBR reactor which makes use of a specific treatment cycle.
The use of selective membrane sensors permitting an online measurement of ammonium concentration (and to a lesser extent, nitrate concentration) is an important element for managing these reactors, permitting an adjustment of partial nitrification conditions (in particular of the Ammonium/Nitrite ratio).
Table 7 below presents a comparison of the needs of the different nitrogen treatment processes:

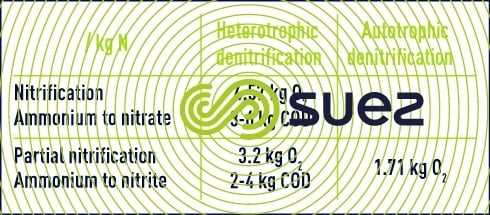

In conclusion, the elimination of nitrogen by Anammox bacteria permits savings to the order of 60% in terms of air supply, does not use biodegradeable carbon and generates little excess sludge. Applied to specific effluents (centrates resulting from the dehydration of anaerobically digested sludge, leachates, industrial effluents) this innovative nitrogen treatment process offers new perspectives for the treatment of urban wastewater: it’s a stake of the future.
Bookmark tool
Click on the bookmark tool, highlight the last read paragraph to continue your reading later
