general
Reading time:principle
Ion exchangers are insoluble granular substances; their molecular structures include acid or base radicals capable of permutating, without any visible change to their physical appearance and without any change in solubilisation, the positive or negative ions that are «fixed» to these radicals, with ions of the same sign found in solution in their surrounding liquid. This permutation termed ion exchange allows us to alter the ionic composition of the liquid undergoing treatment, without modifying the total number of charges that exist in this liquid prior to the exchange.
Originally, ion exchangers consisted of earth pigments (zeolites) and then synthetic mineral compounds (silicoaluminates) followed by organic compounds, the latter now almost exclusively being used under the name of resins. These materials are supplied either as granules or, in most cases, as beads.
We have the resin backbone and the active sites grafted onto this backbone. At present, there are three types of industrial backbones: polystyrene, acrylic or formophenol types. The first two are produced by polymerization (with the formation of beads) and the last by condensation (with the formation of granules). Polymerised backbones are reticulated by an agent such as divinylbenzene. A high level of reticulation will enhance resistance to both mechanical stress (pressure drops) and to osmotic stress (linked to the altered ionic form).
Gel type resins have a homogenous structure.
Macroporous type backbone resin porosity is obtained by adding a foaming solvent. The resulting macropores create a break in the crystalline structure that becomes opaque to light. Macroporous resins are heavily reticulated and have a better organic matter adsorption and desorption capacity.
Active cationic or anionic sites are then grafted onto the backbone to produce acids and bases; their strength will depend on the radical that is fixed (HCO2–, HSO3–, NH3+…).
By convention, a resin that has anionic grafted sites is called a cationic resin because it is used to exchange cations … and vice-versa and this resin is designated R-Ca or R-Na depending on whether it is charged with Ca2+ or Na+ ions.
ion exchange mechanism
applying a softening type reversible reaction


As in the case of any chemical equilibrium, this reaction is governed by the law of mass action, the reverse reaction applying to the exchanger’s regeneration.
If the liquid to be treated is placed in static contact with the exchanger, the reaction will cease as soon as the liquid and the resin achieve equilibrium; however, a greater or lesser ionic leak will almost always persist for the ion that we wish to fix.
Laws governing reversible ion exchange: for each reaction involving two ions A and B of the same sign, we can produce a graphic illustration of the equilibrium achieved between the respective concentrations of A and B in the liquid and in the ion exchanger (figure 75).

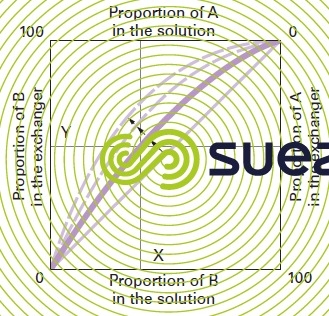

At equilibrium, we can see that for a concentration B equal to X% in the solution, the exchanger will saturate up to a Y% concentration. When both ions A and B have the same affinity for the exchanger, the equilibrium curve will be a diagonal across the square. The more marked the exchanger’s preference for ion B, the more the curve will move in the direction of the arrows.
The shape of the curve, for a given 2-ion system, depends on a number of factors: nature and valency of the ions, affinity for the exchanger.


As discussed above, merely bringing the exchanger and the liquid in contact in the tank would lead to a point on the curve where stabilization would occur.
If we seek to pursue the treatment until we achieve major elimination of one ion to the detriment of the other, we will need to gradually shift the equilibrium by causing the liquid to percolate through a series of exchanger layers, each containing fewer ions to be fixed, in order to follow the equilibrium curve until it comes close to zero concentration for the unwanted ion.
If we start with an exchanger layer that is totally of the A type and if we cause a liquid charged with B ions to percolate through this layer, the successive equilibriums achieved between A and B will result in a series of isochronous concentration curves that have been given in figure 76-a) for two similar affinity ions and in figure 76-b) where the exchanger has a far greater affinity for the B’ ion than for the A’ ion. The «break point» will be reached when the isochronous curve leaves the right hand vertical axis (positive B concentration in the liquid outlet curve). At that point, these curves will appear as seen in figure 76-c).

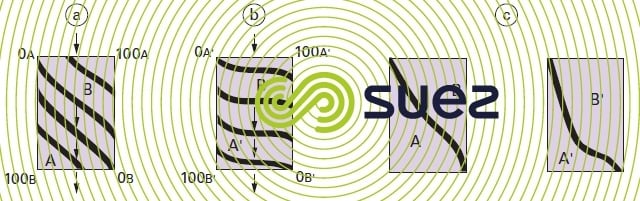


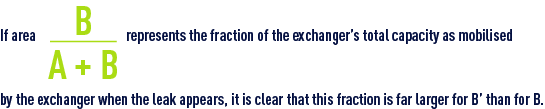
This feature is also apparent in the treated liquid and shown up by the shape of the «saturation curves» (figure 77).

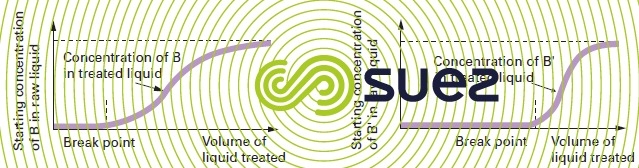

The shape of these curves depends not only on the static equilibrium curve mentioned above but also on the «exchange kinetics» between the liquid and the exchanger, kinetics that involve the penetration of solutes into the exchanger, governed by the laws called «the Donnan membrane equilibrium» laws.
These are highly complex phenomena; they involve both the level of ion dissociation and concentration, temperature, the nature of the exchanger-liquid interface and the penetration kinetics into the solid that is this exchanger.
An ion exchanger’s total capacity, i.e. the total number of equivalents available per litre of exchanger, only has a relative practical value; the industrial factor that has to be taken into account is the «useful capacity» established on the basis of isochronous graphs or saturation curves described earlier.
In practice, the quality of the liquid treated depends exclusively on the saturation state of the final layer crossed by this liquid, regardless of the state of the preceding layers.


Where «fixation» goes from left to right and «regeneration» from right to left, the state of the exchanger should be examined at the start of the fixation cycle that follows a regeneration cycle. It is quite clear that at the start of the fixation cycle, the quality of the treated water, characterized by the ion leakage, depends primarily on the degree of regeneration undergone by the exchanger discharge layer.
applying an irreversible reaction
This involves fixing of strong acid by an very alkaline anion exchanger (neutralization reaction) :


The reverse reaction (hydrolysis), is virtually non-existent; the exchange is total and can be achieved through both static contact and percolation. In this case, we can have zero ion leakage providing that there is sufficient contact time between the water and the resin …. Resin regeneration in the chloride form is only possible with a strong base :


Equilibrium reactions that produce an insoluble compound can be likened to this category of exchange. For example, if we treat seawater in an exchanger that is saturated with silver ions, we obtain :


AgCℓ being insoluble, precipitates. Under these conditions, according to the laws of Berthollet, the equilibrium will shift completely and the reaction is complete, even in static contact systems.
Both of the above types of reaction can be used :
- to remove one or more unwanted ions from the treated liquid;
- to select and concentrate one or more ions in the exchanger; these ions will subsequently be found in their purified state and concentrated in the regeneration liquid.
using a complex anion that has previously been fixed
This complex ion is likely to induce secondary reactions such as oxidation-reduction phenomena affecting the ions in the water or treated liquid, without this ion forming a solution in the liquid.
E.g. dissolved oxygen fixing by oxidizing a sulphited anionic resin (R-NH3-S03H) to produce a sulphated resin (R-NH3-SO4H).
other applications
Apart from ion exchanges, resins can be used as catalysts (e.g. acid) or as adsorbents or colour removers …
regeneration methods
In softening and demineralization processes, the end of the cycle occurs when the saturation curve matches the one shown in figure 76-c) (compounds A’ and B’). At least with regard to the upper layers, we can then assume that the ion exchanger is almost saturated with B’ ions and in equilibrium with the B’ concentration in the incoming solution.
Regeneration takes place by percolating a concentrated A’ ion solution, either in the same direction as saturation (co-current regeneration) or in the opposite direction (countercurrent regeneration).
co-current regeneration
During this operation (figure 78), the concentrated A’ ion solution is first put in contact with the ion exchanger layers that are B’ ion saturated; these B’ ions are then conveyed to the ion exchanger layers that are less saturated and where they find conditions that are favourable to their fixing; these ions will mainly be the A’ ions that remain in the bottom layers of the column and that are eluted at the start of regeneration.

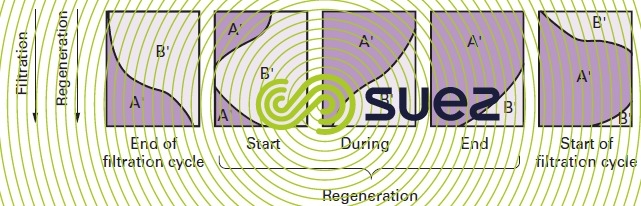

Finally, if the amount of regenerant is limited, the B’ ions will not be totally eluted from the ion exchanger and the lower layers will not be completely regenerated.
Therefore, during the following cycle, the B’ ions will undergo self-regeneration by the A’ ions that have been moved out of the upper layers.
Therefore, it would seem, that in order to achieve a satisfactory ion exchanger regeneration, it will almost certainly be necessary to use an amount of A’ regenerant that is twice the A’/B’ stoichiometric ratio.
countercurrent regeneration
The phenomena change when regeneration reagents are injected from the bottom upwards; in effect, in this case, the concentrated A’ ions first meet resin layers that only have a light B' ion concentration and elution thus takes place under good conditions; additionally, in the upper, saturated, layers, the B' ions cannot refix themselves (figure 79).

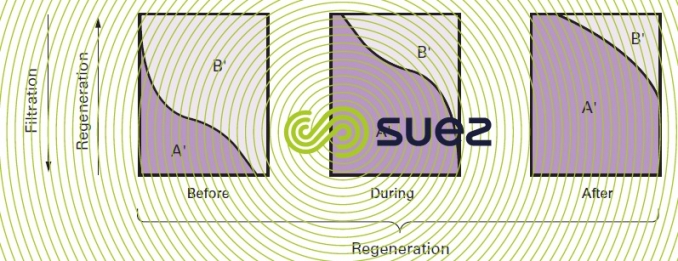

We need to emphasise two important advantages of countercurrent regeneration :
- improved performance and, therefore, lower reagent consumption for the same useful capacity;
- improved treated water quality, the high excess of reagent being used to regenerate the discharge layers.
ion exchange vocabulary
The capacity (or exchange potential) of an exchanger: this is the quantity of ions likely to be fixed per unit of volume or per unit of weight. The capacity is expressed as the equivalent or in French degrees per unit of weight or of volume of compacted resin.
We have :
- the total capacity which is the maximum quantity of exchangeable ions and which is characteristic of a given resin;
- the useful capacity which is the usable fraction of the total capacity and which depends on the hydraulic and chemical conditions prevailing in each application case.

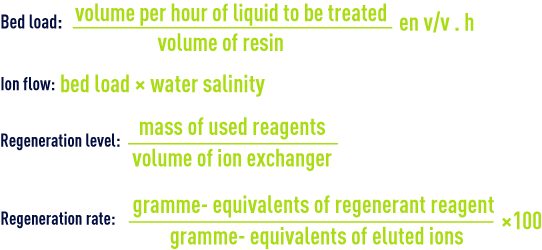
This ratio will always be equal to or better than 100% (100% referring to the Stoichiometry).
- Ion leakage : concentration of the ion to be fixed and that remains in the treated water. It is expressed in mg · L–1, mg · L–1, milliequivalents/litre, sometimes as a percentage in relation to the influent.
- Breakdown : maximum permissible ionic leak requiring suspension of the production cycle.
- Attrition : mechanical wear suffered by the exchanger beads as they are used.
Bookmark tool
Click on the bookmark tool, highlight the last read paragraph to continue your reading later
